
Synthetic microbes are nonnaturally occurring species of bacteria and viruses that can reproduce on their own. A key group involved in the development of these synthetic organisms is the J. Craig Venter Instistue. To begin to engineer such a species, the team first copied the genome of a bacterial species, and then transferred it to a computer. In 2003, JCVI researchers created a synthetic version of the bacteriophage, PhiX 174, and the synthetic genetic code was then transplanted into another bacterium whose genome was removed.
In 2015, two research teams led by Yale bioengineer Farren Isaacs and Harvard geneticist George Church showed that E.coli could be genetically modified to incorporate synthetic amino acids into essential proteins. When the bacteria are not fed the amino acids, they cannot produce these essential proteins, and so they die. Following this strategy results in bacteria that are very unlikely to survive without support from scientists and requires intensive engineering of the bacterial genome.
Controlling Synthetic Microbes
Synthetic Microbes:Microdefenders
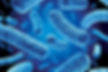
(P4.6)
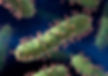
(P4.7)
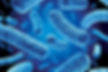
(P4.6)
The practical applications of synthetic microbes are endless: from developing sustainable energy, to designing highly sensitive microbes that could potentially sense the presence of pollutants, toxins or explosives like TNT in warzones, to treating intestinal disorders-- the list goes on and on (4.27, 4.28).
Microbial Kill Switches: Controlling Synthetic Microbes
Two synthetic gene circuits allow researchers to keep genetically engineered microbes alive under specific conditions, and to kill them when they are no longer needed to function. The circuits, described could help pave the path to safe diagnostics, therapies, or environmental remediation strategies that rely on genetically engineered bacteria.
James Collins, an MIT synthetic biologist, began to design these synthetic gene circuits, also known as “kill switches” after becoming interested in using genetically engineered microbes for diagnostic and therapeutic purposes. But with genetic modification comes the concern that scientists will create new and uncontrollable species that will permanently alter the environment or endanger human health.

(P4.8)

(P4.9)

(P4.8)
First, Collins and his team generated a kill switch called Deadman. In this kill switch, a researcher must feed bacteria a substance called anhydrotetracycline at all times, or else the microbes will express a toxin and self-destruct. Other versions of the Deadman circuit can be designed to degrade essential proteins in the absence of anhydrotetracycline.
A second kill switch, called Passcode, similarly requires that researchers maintain a specific environment for cells lest they express a toxin. Passcode also requires a combination of input molecules for cells to survive. The system relies on hybrid transcription factors. Each factor contains one component that recognizes a specific DNA sequence, and one component that is sensitive to certain small molecules. For example, one hybrid transcription factor, factor C, turns off the expression of a toxin within the bacteria. Two other hybrid transcription factors, called factors A and B, suppress expression of factor C. However, specific small molecules can keep A and B from interacting with C. Another small molecule could prevent C from repressing the toxin. Therefore, to keep the bacteria cells alive, the researchers must provide them with two small molecules that keep factors A and B aligned, and make sure not to give them a third small molecule that will interfere with C. It is like solving a passcode puzzle, essentially (4.8).
Watch a news interview with MIT's Christopher Voigt on GMO Kill Switches, provided by RT
(V4.3)

(P4.10)

(P4.10)