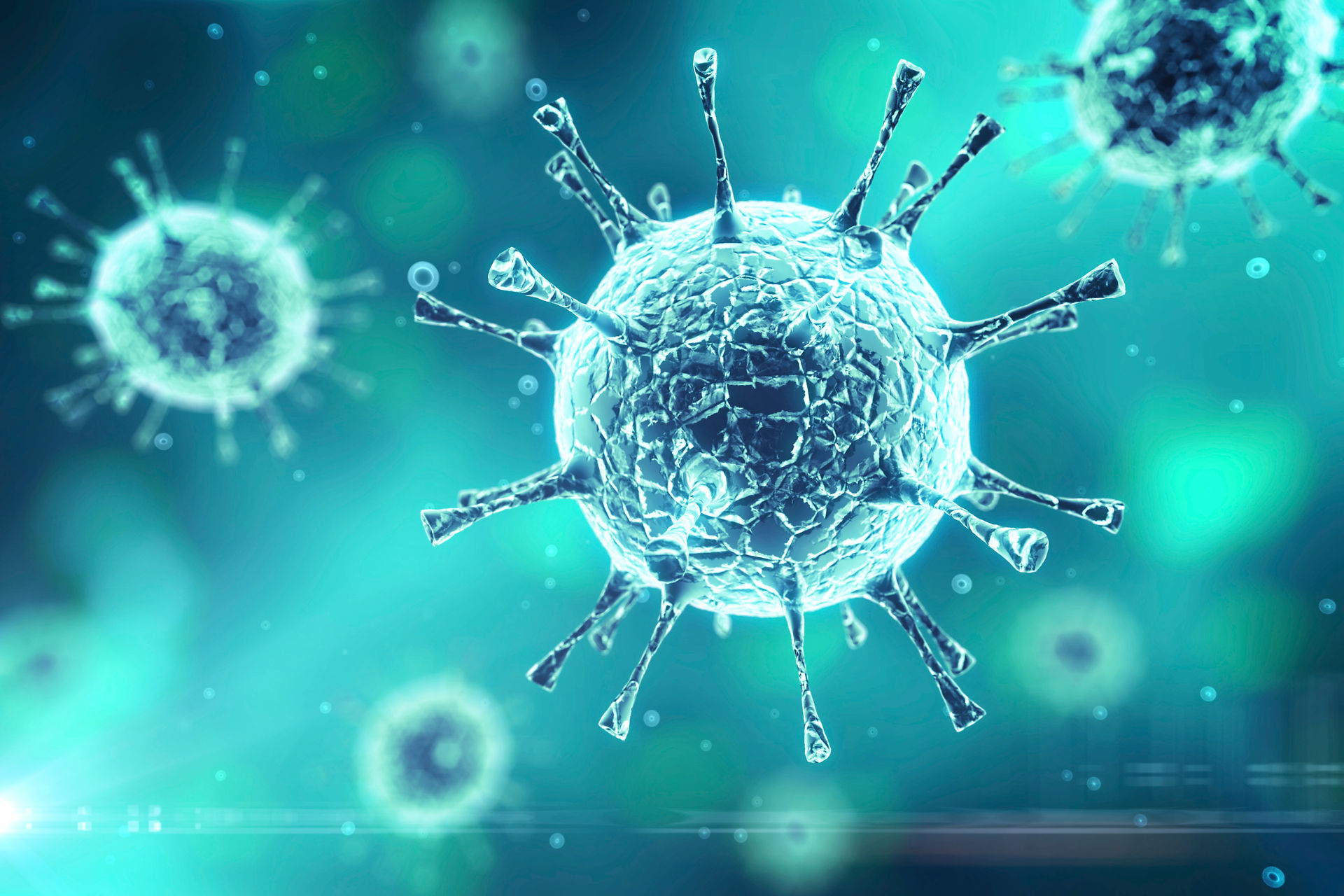


(P5.27)

Design and Modeling
Abstraction
Applying Engineering Principles to Biology
What is Synthetic Biology?
In 2000, Chemical Engineering & News defined Synthetic Biology as
“using the synthetic capability of organic and biological chemistry to design nonnatural, synthetic molecules that nevertheless function in biological systems (5.1)"
Though part of this definition is still applicable today, Synthetic Biology groups have taken to a more practical definition, as SynBerc describes Synthetic Biology as
“making biology easier to engineer (5.2).”
What does DNA have to do with it?
In other words, Synthetic Biology is an approach to engineering new technologies to “build” new biological organisms for useful purposes. This includes the design and building of new biological parts, as well as the re-design of existing biological devices and systems. Synthetic Biology is divided into two major areas: complete Genome Engineering, and small systems and devices engineering. But to be clear, synthetic biology is not necessarily genetic engineering nor is it bioprocess engineering. It is, however, an approach to be able to use these aforementioned technologies. As Stanford Synthetic Biologist Drew Endy states, "Synthetic Biology isn’t about what you make, it is how you make it.”
In a way, it is genetic engineering, but reengineered (5.3).
Over a relatively short amount of time in the scope of science, synthetic biologists have attempted to piece together a coherent definition of synthetic biology; however, doing so has proven a difficult task, because it is a field that is not concrete and ever-evolving to involve more applications and techniques.
DNA, or deoxyribonucleic acid, is the primary sequence and material of synthetic biology.The double-helix structure that is the hereditary material in humans provides genetic instructions used in the development of all organisms; it is what makes each organism unique. The information in DNA is stored as a code consisting of four different chemical bases: Adenine (A), Cytosine (C), Guanine (G), and Thymine (T). To put the importance of these bases into perspective, it must be noted that human DNA consists of about 3 billion bases! The order, also known as the sequence, of these bases provides information that is available to build and maintain an organism. Think of these bases as letters of the alphabet-- they form information for constructing organisms much like letters can combine to form words and sentences (5.4).
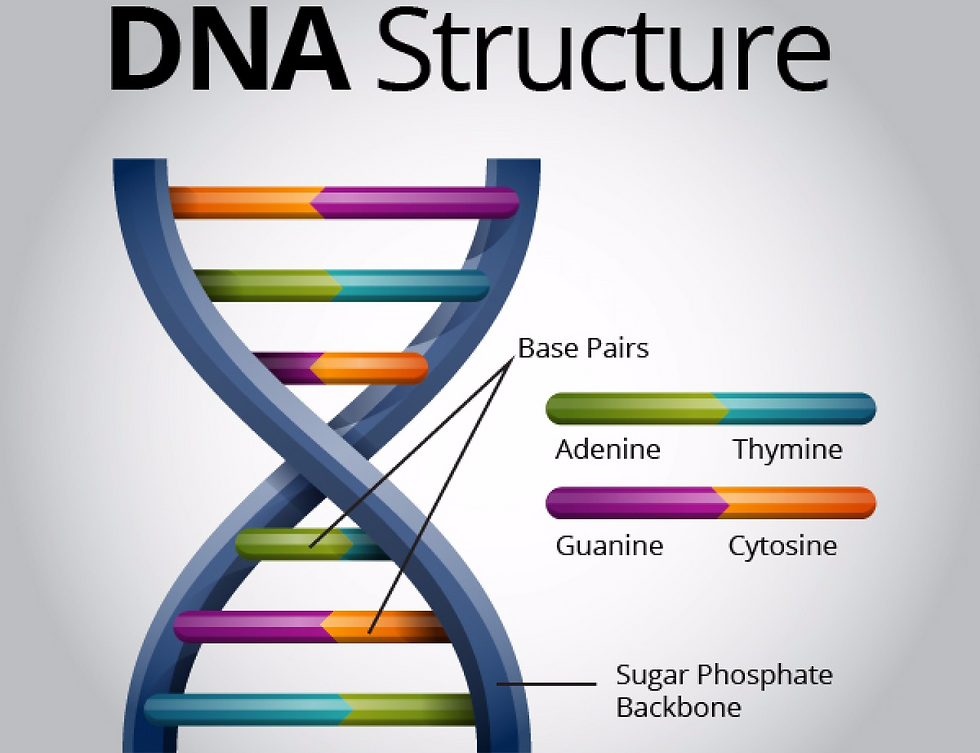
(P5.1)

(P5.2)

(P5.5)
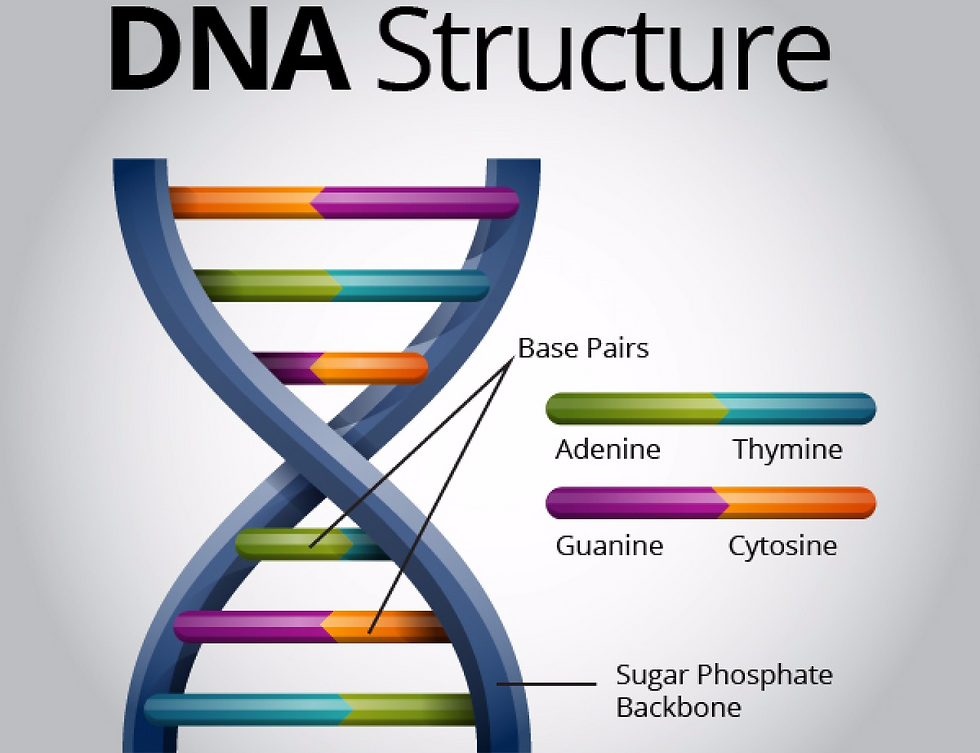
(P5.1)
Did You Know...
Human DNA consists of about 3 billion nucelotide bases? And If you could type 60 words per minute, eight hours a day, it would take approximately 50 years to type the human genome!
What about Chromosomes?
In eukaryotic (plant and animal) cells, Chromosomes are linear thread-like structures located inside the nucleus of the cell; however in bacterial cells, chromosomes tend to be found in single, circular loops. Though data exhibited the former facts, it has become evident that not all bacteria have a single circular chromosome: some bacteria have multiple circular chromosomes, and many bacteria have linear chromosomes and linear plasmids, which are small, circular, double-stranded DNA molecules that are distinct from a cell's chromosomal DNA. Often, the genes carried in plasmids provide bacteria with genetic advantages, such as antibiotic resistance, but they are nonessential and replicate on their own (5.5).
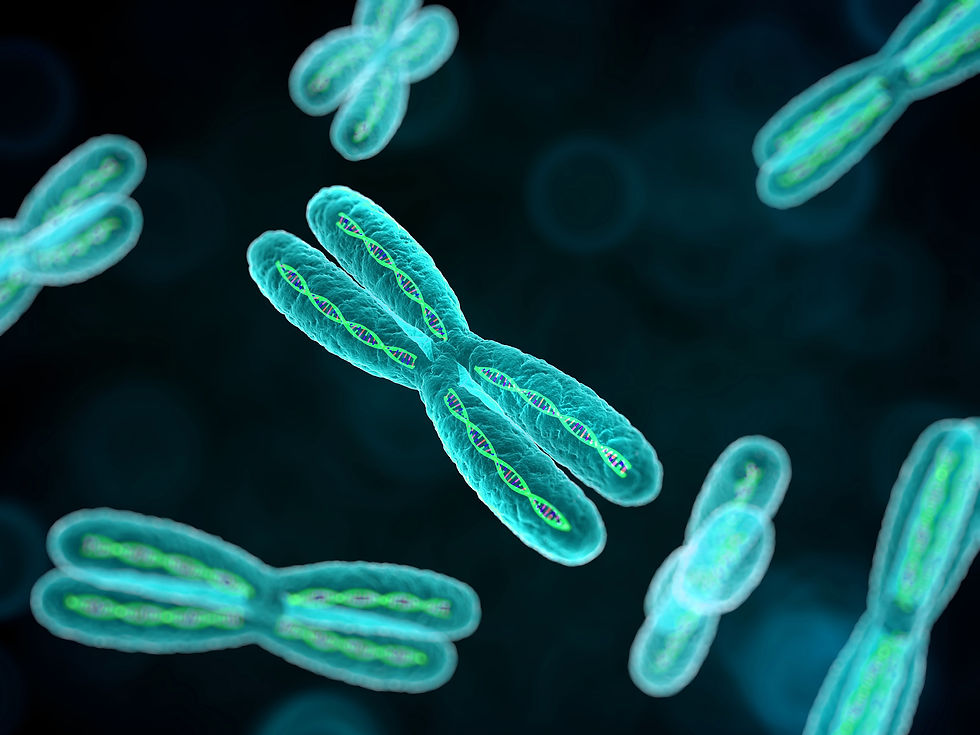
(P5.5)

(P5.6)
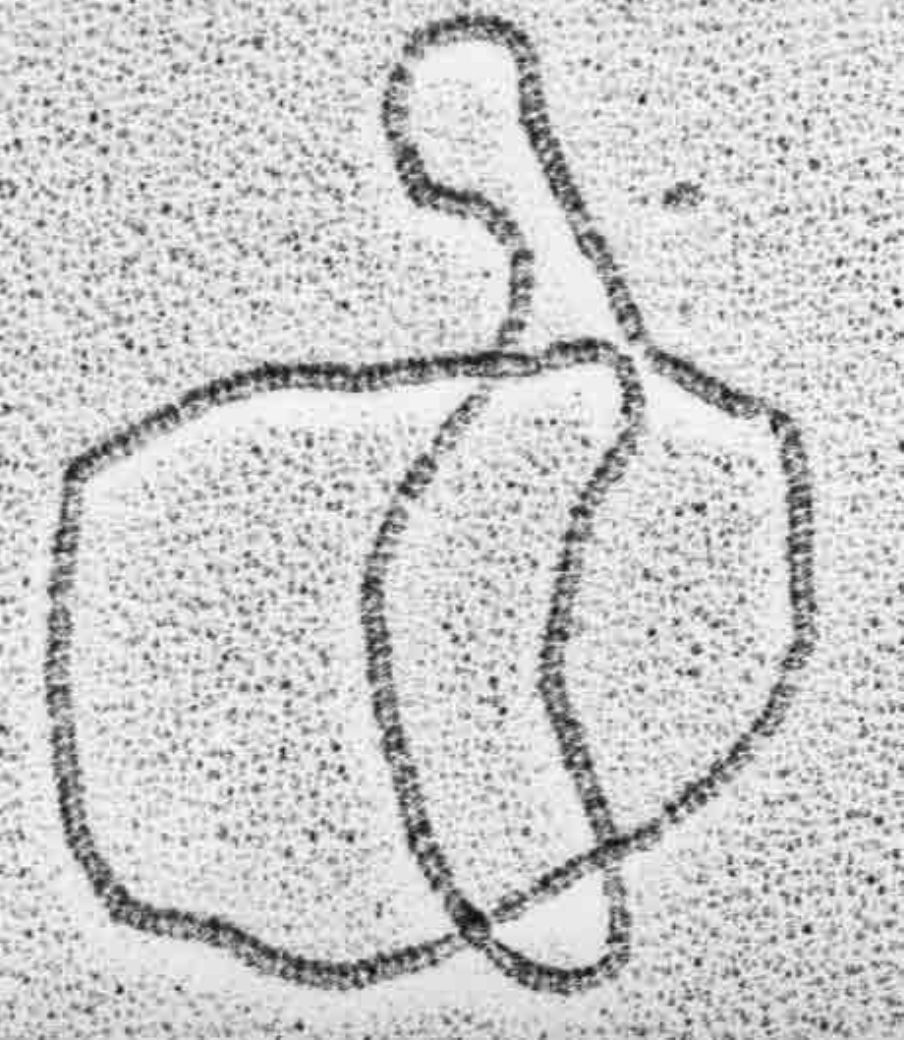
(P5.7)
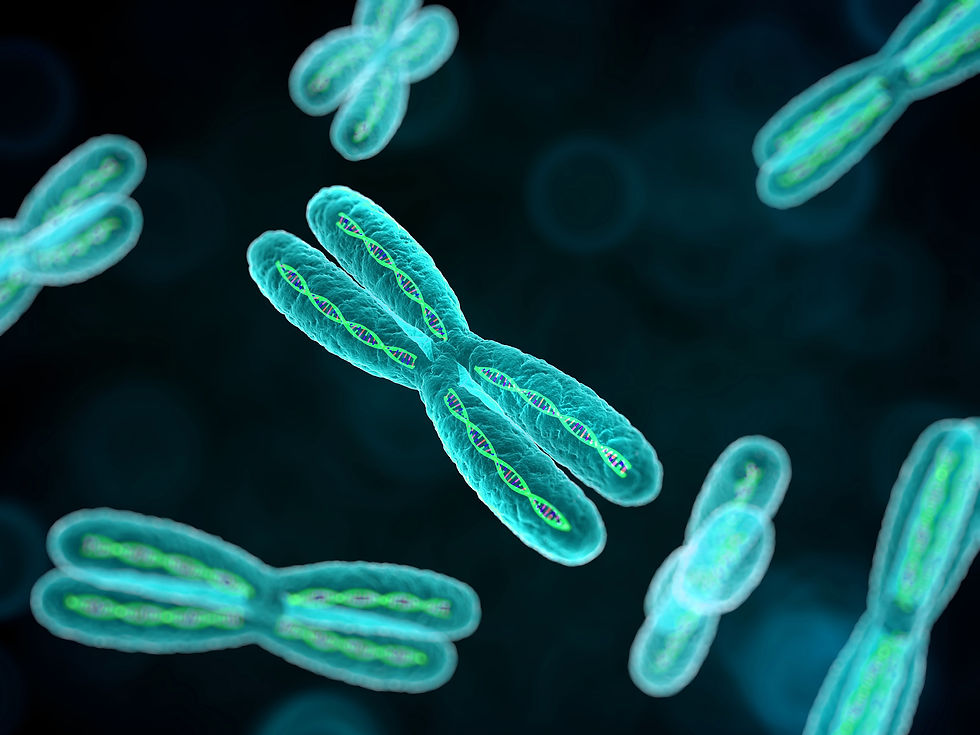
(P5.5)
Experimental evidence for multiple chromosomes and linear chromosomes initially came from studies using pulsed field gel electrophoresis (PFGE), an approach that uses alternating electric fields to separate large DNA molecules on an agarose gel. Subsequently genome sequencing projects have added to the list of bacteria with multiple or linear chromosomes and plasmids (5.5).
DNA's Role in Synthetic Biology
So, how does all that DNA fit inside a cell?In humans, unique structure of chromosomes keeps DNA tightly wrapped around proteins called histones. Without such packaging, DNA molecules would be too long to fit inside cells. For example, if all of the DNA molecules in one human cell were unraveled from their histones and placed side-by-side in a row, they would stretch up to 6 feet. In bacteria, this compaction involves DNA binding proteins that help to form initial loops followed by coiling of DNA. Think of bacterial DNA coiled like a rubber band that has been repeatedly twisted (5.6).
In synthetic biology, DNA is a type of part: the simplest, most basic unit involved in the design of synthetic biological systems. Parts can be either DNA, RNA, or protein molecules that encode and/or carry out a defined biological function – such as binding to another molecule or catalyzing a reaction. Parts can be combined to create devices that carry out more complex defined human functions. Devices can combine to form “device families” that are designed for use in consolidation with one another. Similarly, devices can combine to form a system, which consists of one or more devices encoding a human defined function (5.7)
Systems
Consists of one or more devices encoding a human defined function
Devices
Combined parts that carry out more complex defined human functions.
Parts
DNA, RNA, or protein molecules that encode and/or carry out a defined biological function
DNA Sequencing & Synthesis of Chasses
Foundational Technology
Concurrent with the first synthetic biology projects was the sequencing of the DNA that constitutes the human genome. ENCODE, the Encyclopedia of DNA Elements, is a project funded by the National Human Genome Research Institute to identify all regions of transcription, transcription factor association, chromatin structure and histone modification in the human genome sequence. As a result of the identification of these important elements, 80% of the segments of the human genome now have at least one biochemical function associated with them.
A genome sequence is essentially a blueprint for the function of the cell, and having a complete genome sequence for an organism is vital for understanding how it can be reengineered to be a chassis system for synthetic biology.
But what is a chassis system?
DNA sequencing is determining the order of nucleotide bases (A,C,T and G) in DNA. While DNA sequencing is essentially like “reading” DNA, genome sequencing would be equivalent to “reading” the entire book of all the DNA found in a human. But the most important impact of genome sequencing on Synthetic biology was made in 1996 and 1997 when the sequencing of the genomes of two model organisms, Escherichia coli (E. coli) and the yeast Saccharomyces cerevisiae were made available. These two organisms are both widely used in biotechnology as well as synthetic biology (5.9).
Synthetic biologists refer to the host cell, the cell that has been introduced with DNA or RNA, as the chassis. In order for an engineered genetic system to function in a chassis, the chassis must supply the cell with energy, supplies for synthesizing proteins and supplies that those proteins will need to use for them to function.The more familiar and formally understood the chassis is, the better it can provide materials for the engineered system, and the better the results of a synthetic biology application will be. By using one chassis primarily, synthetic biologists are simplifying the complex nature of the emerging field of synthetic biology. A standardized chassis allows engineers from many labs across the world to compare results, much like a standardized unit system that is available in the scientific world (5.10)
In the case of synthetic biology, a typical chassis that is commonly used is the intestinal bacterium E.coli, because it is well known in that its complete genome sequence is logged and available to access on online databases. E.coli is also easily and quickly grown in simple media, so synthetic biologists can have a batch of new chasses seemingly overnight. E.coli can also be frozen for up to 10 years, which is imperatively important to synthetic biology because it allows the storage of DNA parts within living cells for long periods of time,without change and without human intervention.Though E.coli is the most common chassis, other types of chasses may also be used, depending on the project; for example, the yeast Saccharomyces cerevisiae, a eukaryotic cell, which is easy to grow. Different chassis have various advantages and limitations when compared to one another; for example, one project might be ideally suited to E. coli and another to S. cerevisiae (5.8, 5.9, 5.10, 5.11).
70% Water
15% Proteins
6% RNA
3% Carbohydrates and Precursors
2% Lipids and Precursors
1% DNA
1% Inorganic Ions
0.4% Amino Acids and Precursors
0.4% Nucleotides and Precursors
0.2% Other Small Molecules
E.Coli Chassis Recipe
Explore the ENCODE Project Below!
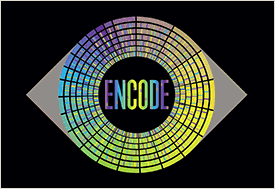
(P5.8)
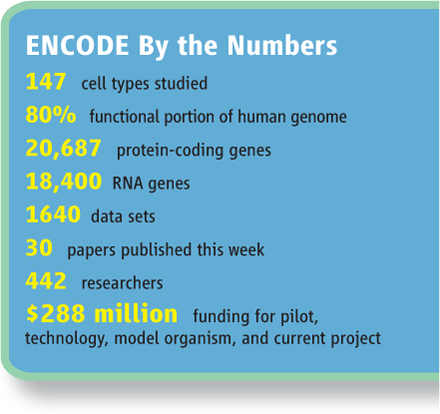
(P5.9)
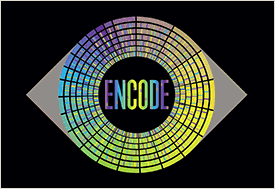
(P5.8)
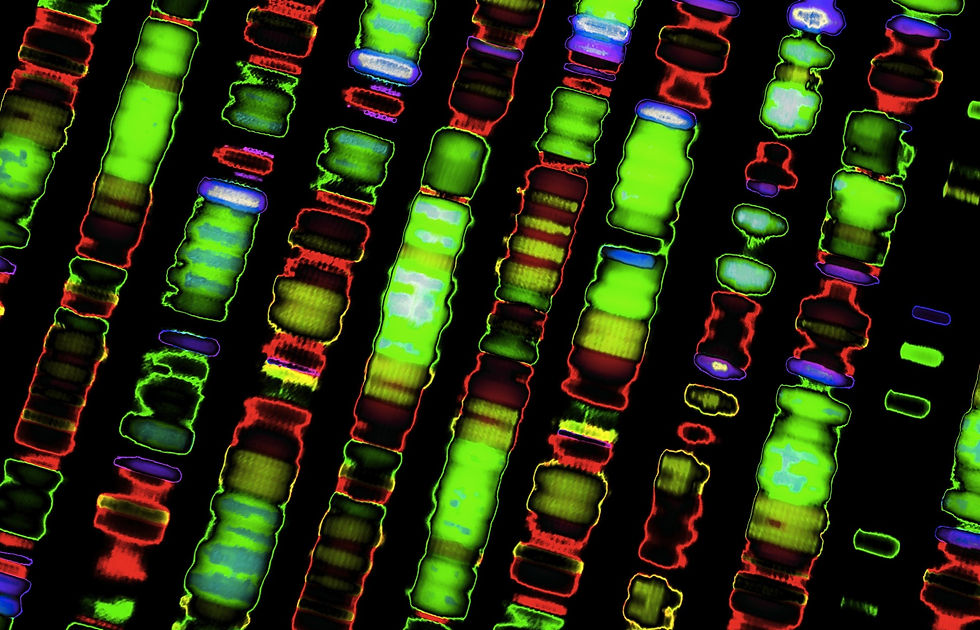
(P5.10)
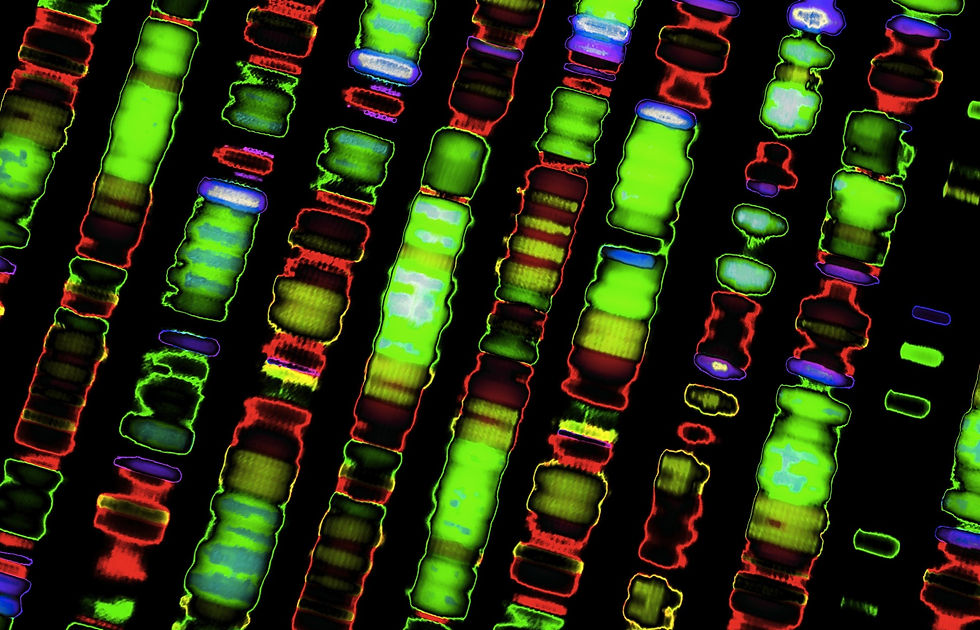
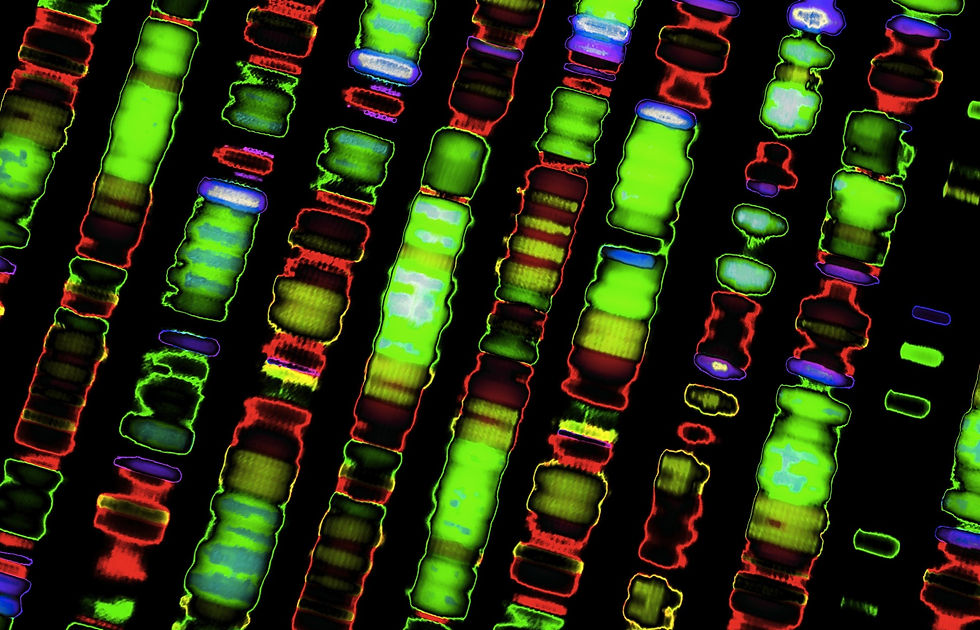
(P5.10)
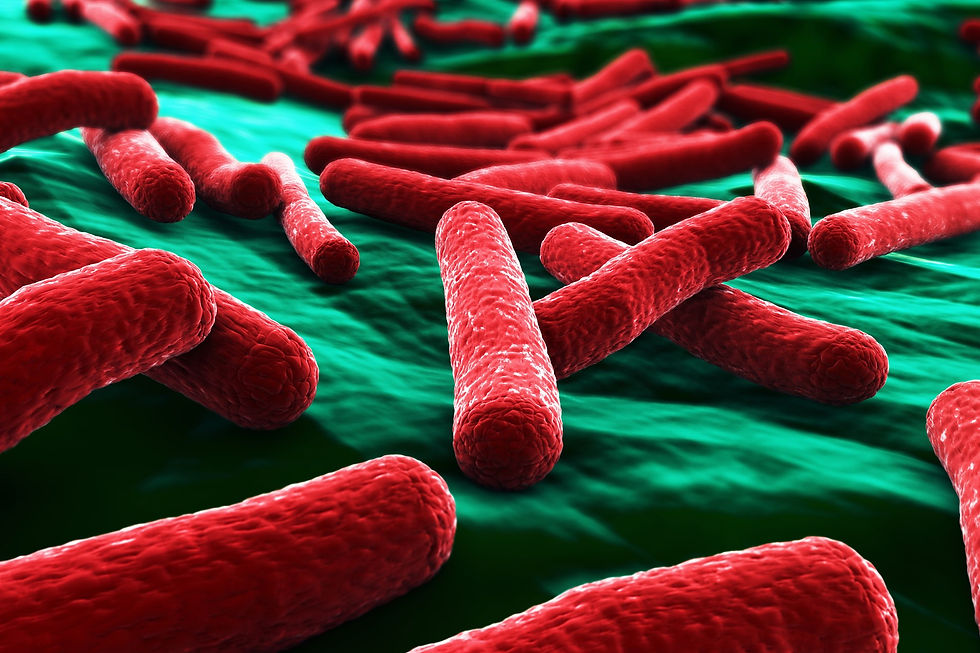
(P5.10)

(P5.11)

(P5.12)
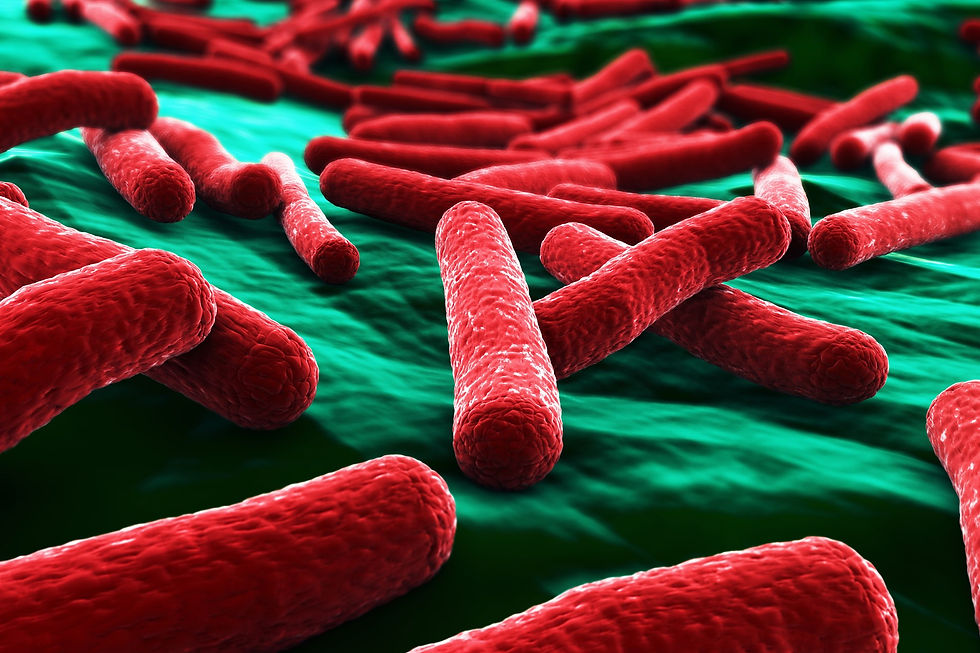
(P5.10)

(P5.13)
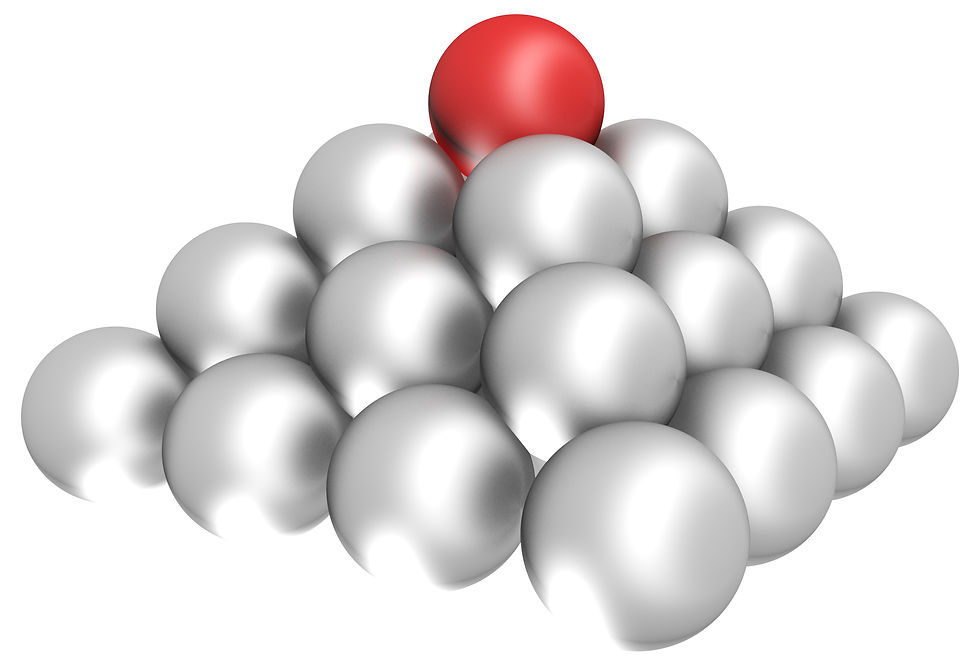
(P5.14)

(P5.13)
Abstraction hierarchies are human inventions that are designed to assist people in engineering very complex systems by ignoring unnecessary details. If the process to design a biological system was to write down the string of nucleotides, it would immediately become extremely difficult, even for experts, to design anything but simplistic systems. If instead, an abstraction hierarchy is specified, it allows the designer of a biological system to ignore some of the implementation details and focus only on the high-level design issues. Engineers in all disciplines take advantage of abstraction hierarchies to design and build complicated systems. Ultimately abstraction means that synthetic biologists are able to use and design complex parts, devices and systems without worrying about every detail of how they work individually, to a molecular level. (Kuldell et al. 5.15).
As with any project, biological or engineering, one must always have a design plan and carry out the plan with a model to test a certain device’s effectiveness and capacity. In Synthetic biology, models allow for better predictions of system behavior prior to fabrication or creation of new systems. Synthetic biology will benefit from better models of how biological molecules, such as enzymes, bind substrates and catalyze reactions, how DNA encodes the information needed for a cell to function as specified, and how integrated systems with multiple components behave. Recently, multiscale models of gene regulatory networks have been developed that focus on synthetic biology applications. Simulations have been used that model all biomolecular interactions in transcription, translation, regulation, and induction of gene regulatory networks, guiding the design of synthetic systems (5.14, Kuldell et al. 5.15).
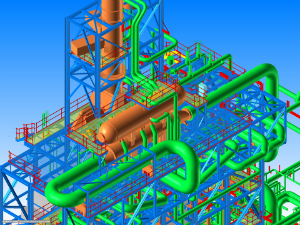
(P5.15)
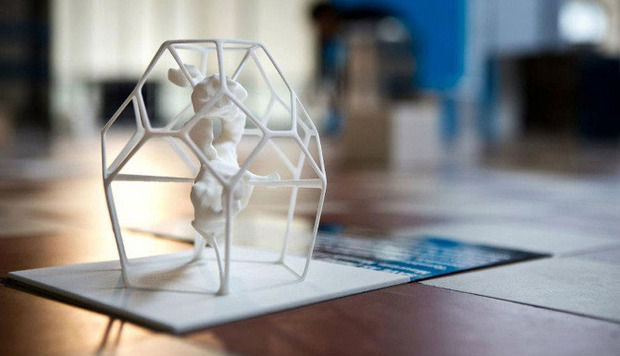
(P5.16)
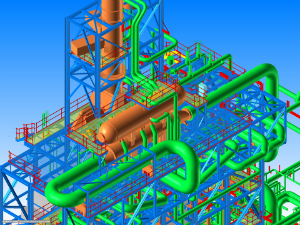
(P5.15)
Characterization, or standardization of standard biological parts is an essential step in enabling construction of more complicated devices and systems based on these parts. A standard method of characterization (or standardization) will better enable sharing of parts between different laboratories, as well as improve our ability to design devices and share data and research about such devices (Kuldell et al. 5.15).
Characterization

(P5.17)
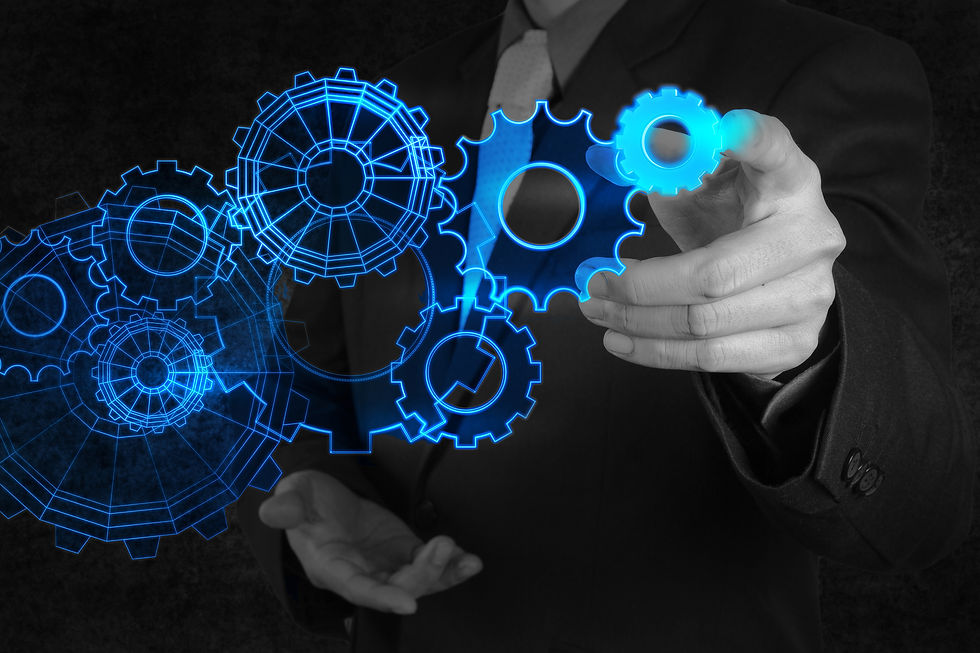
(P5.18)

(P5.17)
Main Intellectual Agendas of Synthetic Biology
The first main agenda is the scientific idea that one practical test of understanding is an ability to reconstitute a functional system from its basic parts. Scientists are actually testing models to better understand how biology works by building systems based on live models, and measuring differences between expectations and results.
Second, the concept arose that, biology is an extension of chemistry and thus synthetic biology is an extension of synthetic chemistry. Current attempts to manipulate living systems at the molecular level will likely lead to a better understanding and novel types of biological components and systems.
Fourth, the idea emerged that biology, the study of life itself, can be used as a technology, and that this biotechnology can be broadly redefined. Within the redefinition of biotechnology is the inclusion of engineering of integrated biological systems for the purposes of processing information, producing energy, manufacturing chemicals, and creating synthetic materials (5.16).
Third is the idea that natural living systems have evolved in order to continue to exist, rather than being optimized for human understanding and intention. This means that living systems have their own agenda, which does not always align with human wants and interactions. Through the redesign of natural living systems, it can become possible to test our current understanding of biological systems while implementing newly engineered systems that are easier to interact with and study to fit ideal human nature.

(P5.21)

(P5.22)

(P5.21)

(P5.19)

(P5.20)

(P5.19)

(P5.24)
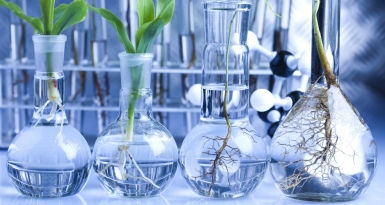
(P5.25)
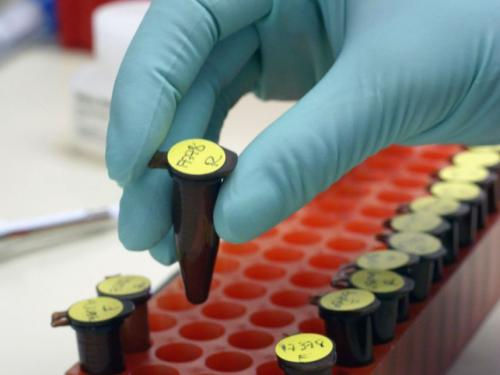
(P5.26)

(P5.24)
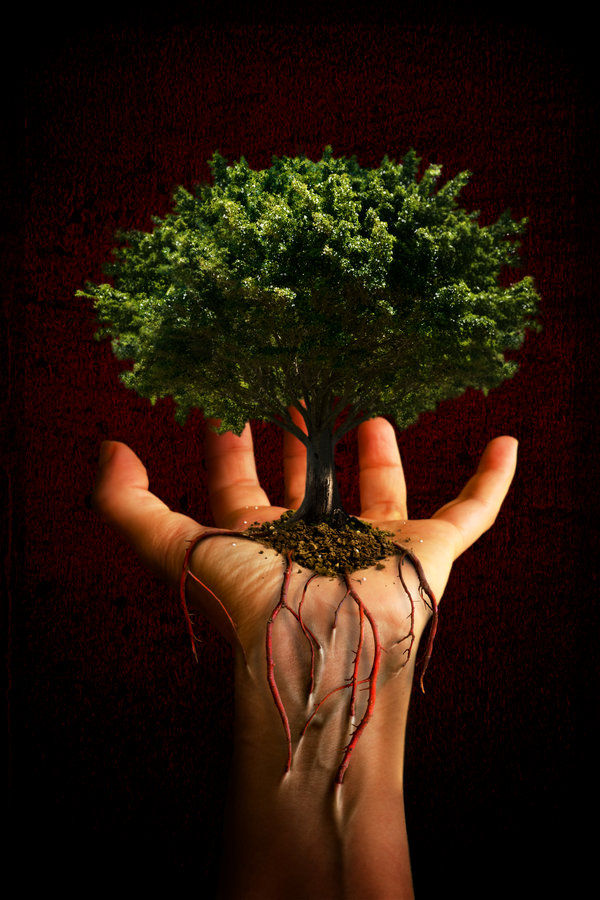
(P5.23)
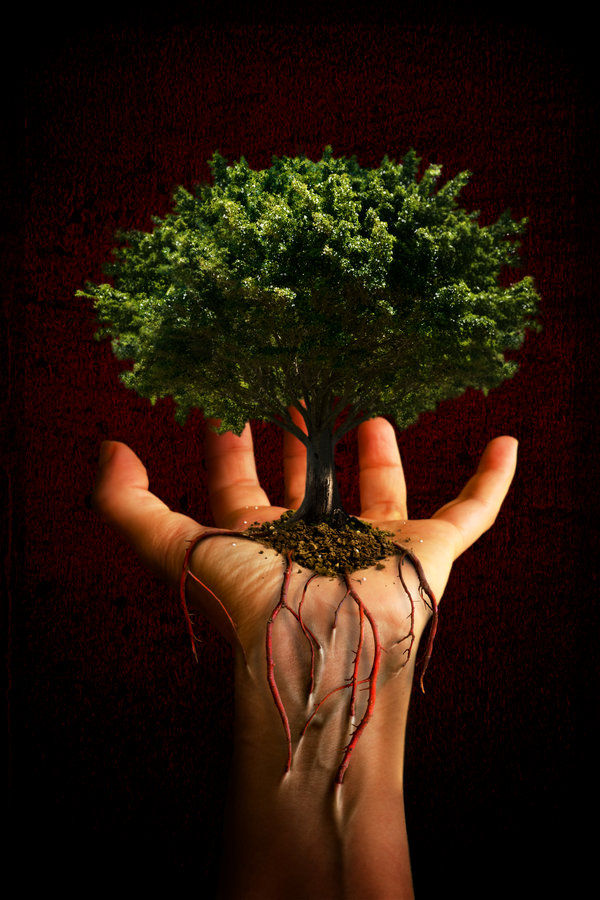
(P5.23)

(P5.24)

(P5.25)
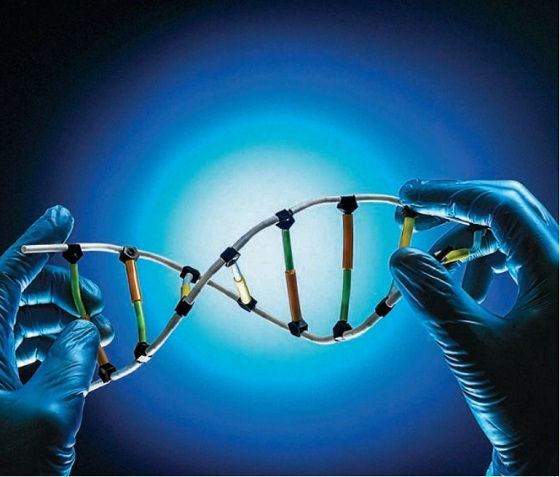
(P5.26)

(P5.24)
(V5.1)
What Makes Synthetic Biology Important?
My people may ask, "what is the use of synthetic biology outside of the lab?" One of the most astonishing phenomena of biology is the fact that cells can replicate themselves. This means that they are their own building factories. Even with only a small amount of necessary nutrients, some bacterial cells can copy themselves and divide in as fast as half of an hour, like the bacterium E. Coli, which is the the "poster child" of synthetic biology. Because of cells' capability to replicate themselves, synthetic biology presents a way for scientists to essentially produce mass amounts of a certain product by enginering cells to replicate themselves to meet demand. This can have implications in fields such as medicine, where drugs need to be created to cure large groups of people.
Another astonishing feature of cells is that these tiny DNA containing molecules contain the biological instruction to be able to carry out
chemical reactions as well as other biological functions. Cells are also able to repair themselves to some degree, and they carry out these functions with a natural, yet extremely high scale of precison, making it hard to replicate a cell's functions in any biofabrication facility. Synthetic Biology has harnessed the ability to re-engineer cells, taking control of the cell's amazing biological capabilities (Kuldell et al. 5.15).
"Though we have certainly come a long way in our understanding of biological systems, we cannot yet build entirely new systems. There is still much to learn about even the most basic biological processes and systems, and synthetic biology provides a powerful new tool in this endeavor, as well." -from Biobuilder
DNA Synthesis
DNA synthesis is the process for the chemical production of DNA strands from scratch and without a pre-existing physical template. In the science lab, chemists have developed alternative methods to produce DNA by chemically adding nucleotides (A,T,C, or G) to a growing nucleotide chain. No matter how DNA is synthesized, it must have the correct sequence. Synthetic biologists, or "biobuilders" as they are sometimes called, are designing new DNA sequences that have never existed in nature When there is no template for scientists to follow, they determine the synthetic DNA's sequence by using digital sequence information. With this technology, synthetic biologists can write new DNA sequences that have never been written before (Kuldell et al. 5.15).
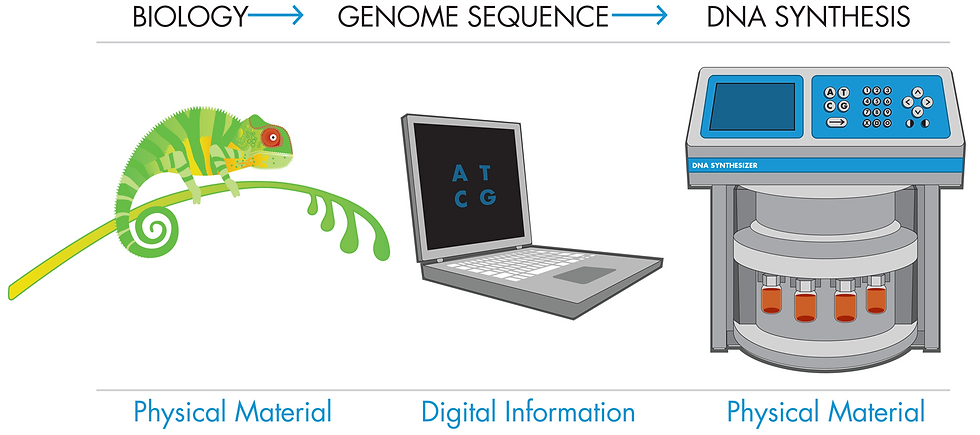
Image courtesy of Biobuilders book by Natalie Kuldell et al.
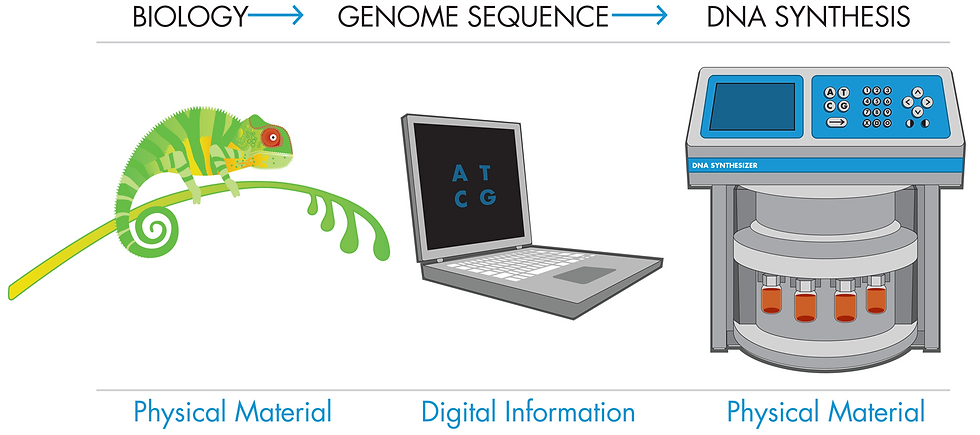
Image courtesy of Biobuilders book by Natalie Kuldell et al.
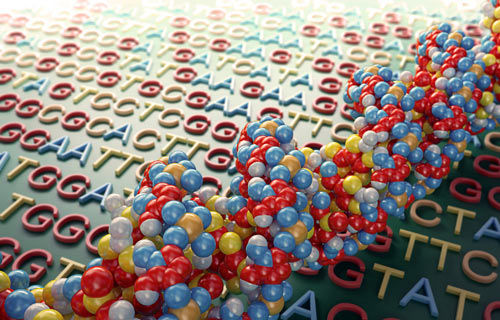
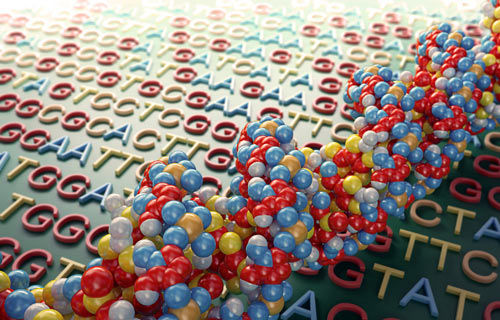

Model of Cell Division

Model of Cell Division

Human Cells

Human Cells